Originally published as:
Maslyn. R. M., 1977, Recognition of fossil karst features in the ancient record: A discussion of several
common fossil karst forms, in Veal, H.K., ed., Southern and Central Rockies Exploration Frontiers: Rocky Mtn. Assoc.
Geologists Guidebook, p. 311-319.
Copyright (c) 1977, 2005 by R. Mark Maslyn - All Rights Reserved
|
|
RECOGNITION OF FOSSIL KARST FEATURES IN THE
ANCIENT RECORD: A DISCUSSION OF SEVERAL
COMMON FOSSIL KARST FORMS
|
by
R. Mark Maslyn
|
|
ABSTRACT
Fossil karst is a general term for those karst features formed in the distant geologic past and now preserved beneath
younger sediments. These features are important indications of periods of uplift of carbonate rock and subaerial
exposure. Additionally, such karst development may alter carbonate rock porosity and influence its reservoir characteristics.
Fossil karst features are widespread in the United States, having developed at various times during the Paleozoic,
Mesozoic, and part of the Cenozoic. The two most notable episodes of karstification, however, occurred in the post-Lower
Ordovician and in the Late Mississippian. Both of these episodes left locally developed karst features including
an erosional karst topography, carbonate soil breccias, filled caves, sinkholes, and residual karst towers over
a widespread area. Silicification accompanied the karsting in some areas, replacing the carbonate breccia fragments
and the carbonate wall rock around some of the fossil karst sinkholes.
Positive features such as karst towers extend into the overlying sediments which fossilized them. Similar features
have served as a base for later reef growth and influenced carbonate sedimentation patterns. The recognition of
such fossil karst towers in the ancient record thus may be of importance in locating areas of potential hydrocarbon
accumulation. |
INTRODUCTION
Recognition of fossil karst or "paleokarst" features in the ancient record is of importance to geologists
in general as marking periods of emergence and subaerial weathering, and to petroleum geologists in particular
as the development of karst features may create secondary porosity in a carbonate unit or limit hydrocarbon migration
by forming stratigraphic traps. Areas where oil production is associated with or limited by fossil karst features
include the Elk Basin field along the Wyoming-Montana border (McCaleb and Wayhan, 1969.), the western Williston
basin (Roehl, 1967), and in western Kansas (Ebanks, et al, 1977).
Fossil karst features formed in several areas of North America at various times during much of the Paleozoic and
Mesozoic and are preserved beneath later sediments. Of these various periods of karst formation the most notable
are the post-Lower Ordovician and the Late Mississippian episodes. Most of the examples cited in this paper will
be taken from this latter period.
Fossil karst is distinct from modern karst formed during the Pleistocene or relict karst formed in the Late Tertiary
under different climatic conditions than prevail today. Campbell(1977), |
in considering at what period to separate fossil karst from modern karst, chose the mid-Tertiary as the dividing
point.
While karst is generally considered in terms of caves and sinkholes, topographically negative forms, it can also
include very striking positive forms such as "towers" which can extend 600 feet above the surrounding
countryside. Such positive features can also he readily identified as fossil karst forms.
The term "karst" will be used in this paper for "an aggregate of the characteristic land forms and
subsurface features produced primarily as a result of the solutional removal of minerals within rock or sediment"
(Quinlan, 1972). What follows are brief, descriptions of several major fossil karst features pre- served and recognizable
in the ancient record. As the descriptions are necessarily brief, they should be considered as representative in
general but slightly variable in specific cases.
CARBONATE THICKNESS VARIATIONS
Karst is most commonly associated with an area having a characteristic erosional topography with some relief Produced
by solution. This relief is of a more, local nature as distinct from a regional thinning across an emergent highland.
When buried by later sediments this erosion surface with its relief is preserved. Thinner carbonates are present
where solution |
|
Page 1
|
|
|
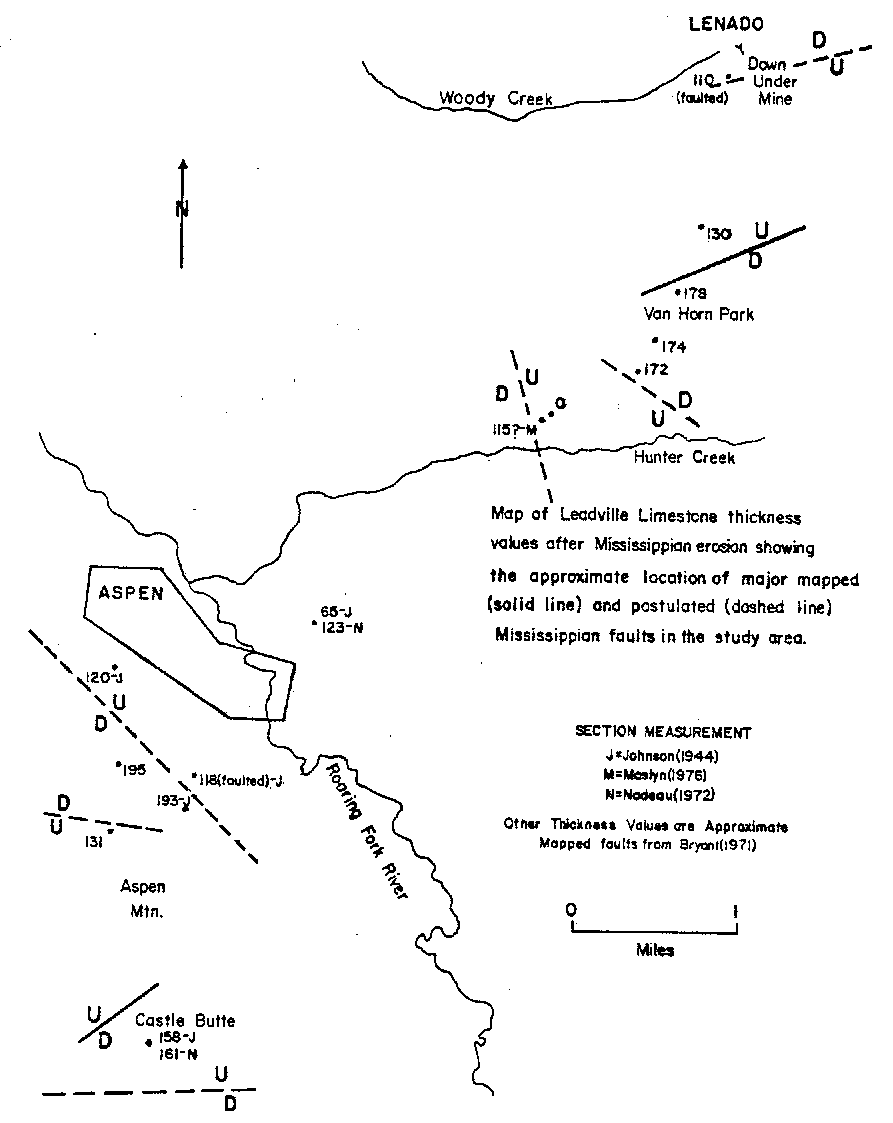
Fig. 1 - Map of-Leadville Limestone thicknesses across horst and graben blocks after Mississippian karst development.
From Maslyn(1976). |
has been greatest and correspondingly thickened sections of the overlying formation occur, filling these eroded
areas.
Dings and Robinson (1957) mapped one such area in section 3 1, T 51 N, R 81 W, in Gunnison County, Colorado, where
pre-Pennsylvanian erosion removed over 500 ft of primarily carbonate rocks. A thickened section of Pennsylvanian
Belden Formation deposited on eroded Ordovician Manitou Dolomite fills this area. A similar example occurs at Reservoir
Hill, 3 miles cast of Aspen, Colorado where 300 ft of section has been removed and the Belden is deposited on the
Manitou Dolomite. In both cases the lower contact of the Belden can be traced laterally as it rises across the
section to rest on the Mississippian Leadville Limestone a short distance from the eroded area.
The most striking example of carbonate thinning, however, occurs across Mississippian-age fault blocks in the Aspen
area (Maslyn, 1976). Here Late Mississippian faulting raised the Leadville Limestone in a series of horst and graben
blocks ranging in size from a few thousand ft to over a mile in width. Solution activity was more intense on the
topographically higher horst blocks than on the lower graben blocks, with a notably decreased resulting carbonate
thickness on the horsts (Fig. 1).
Abrupt changes in carbonate thickness due to small sinkholes or fossil karst towers are treated in later sections.
SOIL BRECCIAS
Although not often considered as a karst feature, a carbonate fragment-rich soil developed on the surface of an
emergent carbonate unit is one of the more common and best preserved records of emergence and solution activity.
The carbonate fragments are variable in size, often in the 2 to 4 in. range, angular to sub-rounded, and contained
in a soil matrix that may be red, brown, or black, depending on local conditions. These fragments |
are derived from the underlying carbonate rock and mantle the uneven topography developing on the surface of the
emergent unit, sometimes collecting in the lower areas and in sinkholes. Early workers often incorrectly referred
to these soil breccias as bedding-plane fault breccias or basal conglomerates.
A well-developed soil breccia occurs in the Aspen area at the contact of the Leadville Limestone and the overlying
Belden Formation. The breccia is composed of angular to sub-angular fragments of the Leadville in black, carbonaceous,
shaly matrix material. Within the breccia matrix are areas of curved slickensides up to 3 feet in length. These
slickensides are the result of fracturing from uneven compaction of the breccia matrix by overlying sediments (Maslyn,
1976).
An analogous breccia occurs along the contact of the Leadville with the overlying red shales and siltstones of
the Molas Formation in the San Juan Mountains of southwestern Colorado. Merril and Winar (1959) have interpreted
the breccia to be a residual soil breccia formed in what may have been a podzol or a laterite dining the Mississippian
emergence of the Leadville
Figure 2 shows a modem carbonate soil breccia forming on the Jurassic Solenhofen Limestone in West Germany.
CAVES
Caves are one of the more easily recognizable fossil karst features and am especially important to economic
geologists as these have localized base-metal mineralization in several carbonate mining districts in the United
States (Walker, 1928, McKnight and Fischer, 1970).
Fossil caves are divisible into two major categories: those that were filled with material in the distant past
and may still be filled or were possibly exhumed in the Tertiary, and those caves that have remained open throughout
their history. The latter group are a minority, having survived both infilling following their |
|
Page 2
|
|
formation and compaction under the pressure of overlying sedi- ments.
Such open caves commonly consist of a few relatively short passageways up to a few hundred ft in length, or may
he iso- lated vugs up to 60 ft across. Both kinds are often lined with dogtooth spar calcite crystals up to a few
in in length. Richards (1955) describes several such vugs in the Mississippian Madi- son Limestone of southern
Montana and Wayne M. Sutherland (personal communication, 1976) has suggested that Eaton's Cave on the east side
of the Bighorn Mountains of northern Wyoming is a pre-Pennsylvanian feature (Fig. 3).
The majority of paleokarst caves are filled. Fill materials include red siltstone or clay, quartz sandstone, or
a carbonate breccia in a clay residuum or soil matrix. Hart (1 956) and Sando (1974) have described several such
caves up to 50 ft wide in the Madison Limestone of Wyoming. These caves have been en- countered to depths of 350
ft below the top of the Madison.
Campbell (1977) suggests that some partially filled caves in
z
Fig. 2 - Photograph of a carbonate fragment rich soil developing on the Jurassic Solenhofen Limestone in West Germany.
Photo by Wilson Herrold. |
southern Montana are of Paleozoic age and that Laramide tec- tonism has tilted the caves and their contained sediments.
Fossil caves also occur in the Leadville Limestone in the Aspen, Colorado area where they have been exposed by
erosion and by mining. The largest cave, over 100 ft in width, is located in Spar Gulch on Aspen Mountain. This
cave formed in the limestone above a carbonate breccia-filled hollow at the unconforrnity between the Redcliff
and Castle Butte Members of the Leadville. Cave development continued at this level until the cave roof failed,
brecciating as it sagged downwards into the void (Maslyn, 1976).
Another fossil cave is exposed in the Down-Under mine at Lenado, 6 mi. northeast of Aspen. The cave is approximately
12 ft across and is filled by a breccia composed of carbonate fragments in brown fine-grained residuum and black
carbona- ceous shaly matrix (Fig. 4).
SINKHOLES
Sinkholes form by two main mechanisms; the failure of cavern roofs, with the collapse extending through the overlying
beds to the surface, and by the solutional enlargement of closely spaced fractures with slumpage into the voids
created along the fractures.
Fossil sinkholes are not always recognized as such in the ancient record as they are seldom completely exposed.
Where erosion or construction work has exposed a sinkhole the contact between the carbonate wall and the sinkhole
filling is abrupt and cross-cuts the carbonate unit, truncating it laterally. Such sinkholes are common in scattered
areas of the Rocky Mountains and elsewhere in the United States.
These fossil sinkholes are distinct from "structural sinkholes" which possess the form of sinkholes but
not necessarily the topographic expression (Cloud and Barnes, 1946). Examples of the latter are described from
the Ordovician Ellenburger Group in Texas by the same authors. The structural sinkholes may represent collapse
of overlying sediments into Ellenburger caverns which survived the episode of karst formation but collapsed under
the pressure of later overlying sedi- ments.
Examples of sinkholes formed during the period of emergence are documented by Keefer (1963), Sando (1974), and
Maslyn (1976, 1977). In northern Wyoming, Sando (1974) notes that sinkholes up to 90 ft deep are developed in the
upper Madison Limestone and filled with angular carbonate breccia fragments in a red siltstone matrix. The matrix
may have the same origin as the red siltstone of the overlying Ainsden Formation. Such sinkholes are occasionally
intersected and exposed by modern caves in the same area. |
|
Page 3
|
|
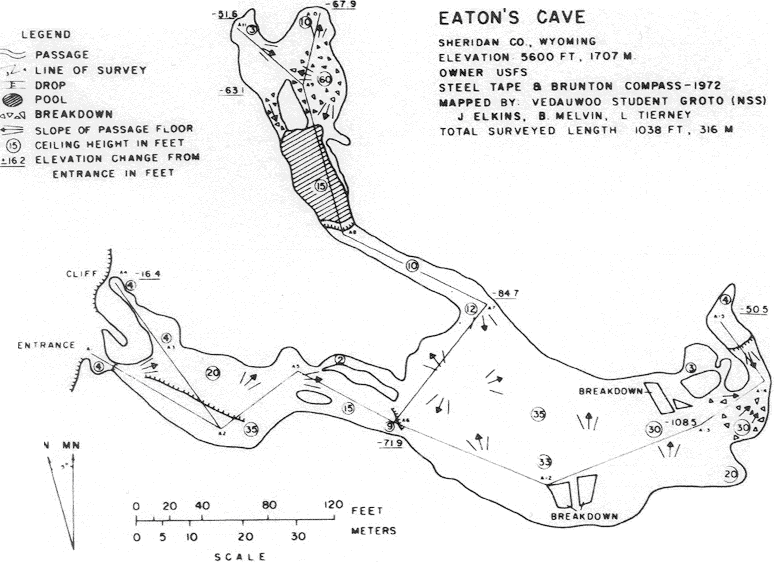 |
Fig. 3 - Map of a possible fossil karst cave in the Mississippian Madison Formation in northern
Wyoming. From Hill et al (1976)
|
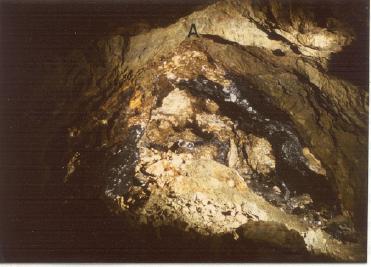
Fig. 4 -View of a fossil karst cave exposed in the Down-Under Mine at Lenado, Colorado. The cave is approximately
12 feet across. The letter "A" indicates the top of the cave. From Maslyn(1976)
Keefer (1963) reported an exhumed fossil karst in the Gros Ventre Range of Wyoming. Here erosion at a high elevation
has exposed over a square mile of filled sinkholes and caves in the upper Madison Limestone. |
In the Aspen area, fossil karst sinkholes up to 250 ft deep and 1500 ft in length are developed in the Leadville
Limestone on Aspen Mountain (Maslyn, 1976). These sinkholes were studied in detail where they are exposed on the
surface and where intersected by mining at the Down-Under mine at Lenado. The sinkhole-filling breccia provided
a favorable host environment for the base-metal-silver mineralization found in the area, several sinkholes having
produced major quantities of ore.
Best exposed of the major sinkholes is the Castle Butte sinkhole, located adjacent to Castle Butte on the upper
slopes of Aspen Mountain. headward erosion of a small valley has exposed the sinkhole in cross-section from the
top of the Leadville Limestone to the base of the sinkhole at the contact of the underlying Dyer Dolomite and Parting
Quartzite for a total depth of 250 ft. The sinkhole is approximately 600 ft long and 350 ft wide with the long
axis oriented slightly west of north and is located adjacent to one of the several block faults common to the area
(Fig. 5).
Within the sinkable are angular fragments of limestone and dolomite derived from the Leadville Limestone and Dyer
Dolo- |
|
Page 4
|
|
mite which contain the sinkhole. Breecia fragments are encased in a brown-tan calcareous siltstone, probably derived
from the residuum left after limestone solution. Individual breccia, fragments range from sand-sized to blocks
10 ft or more across. Many of the larger blocks are oriented subparallel with the bedding in the nearby limestone.
The nature of the breccia lends itself to strong vertical jointing with resulting breccia "ribs" 15 ft
or more in height extending vertically from the sinkhole. Remnants of black Belden shale are also found as sinkhole
filling (Fig. 5). These remnants were deposited in the then-as-yet unfilled sinkhole and contain no carbonate breccia
fragments.
In the Down-Under mine at Lenado, mining has intersected a fossil karst sinkhole of similar dimensions to the Castle
Butte sinkhole. This sinkhole is filled with an interlayered and sometimes intermixed sequence of brown and black
matrix |
carbonate breccia. Individual layers of breccia in black Belden matrix reach 30 ft in thickness in the mine workings
and greater amounts where intersected by drill holes.
Fossil sinkholes are also exposed in the Molas Lake area of the San Juan Mountains in southwestern Colorado (Maslyn,
1977). These sinkholes are located adjacent to fossil karst towers and range up to 300 ft in length. As these sinkholes
appear to have been totally exhumed, no sinkhole-filling breccia is present. The topographic form of the sinkholes
is readily discerned, however, and most are now occupied by ponds and small lakes.
SILIFICATION
The association of silica with unconformities and fossil karst has long been recognized. Leith (1925) in an excellent |
|
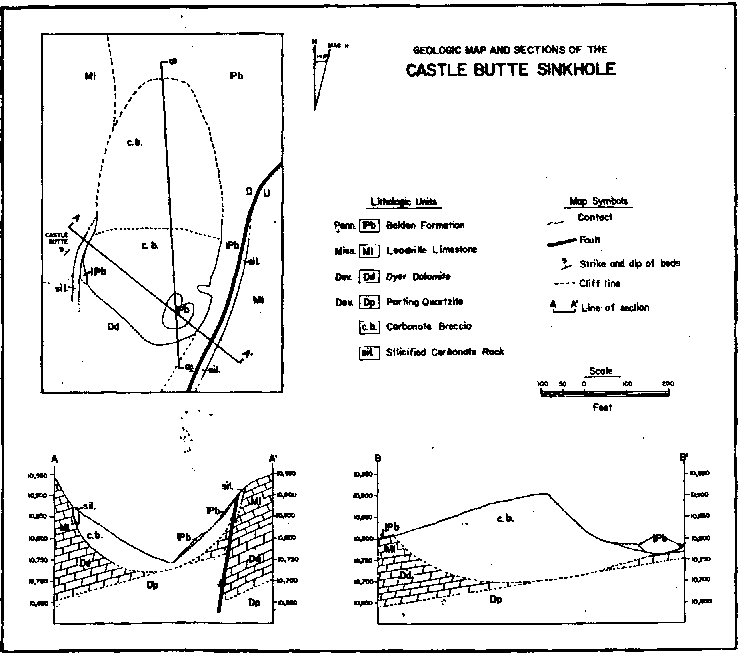
Fig. 5 - Geologic map and cross-section of the well-exposed Castle Butte sinkhole, Aspen Mountain, Colorado. From
Maslyn (1976). |
|
Page 5
|
|
paper on the subject notes that limestones and dolomites are the "most susceptible to siliceous alteration."
In his discussion of silicified unconformities in the Paleozoic rocks of the Mississippi Valley, he states that
"the surface silicification locally extends down along fissures, caverns, and water courses deep within the
limestone formations." Burdon and AI-Sharhan (1968) have also described silicification present on the upper
surface of the paleokarstic Damman Limestone aquifer in Kuwait.
Spurr (1898) first noted the widespread occurrence of silica-replaced carbonate rocks in the Aspen, Colorado area,
giving the name "jasperoid" to those rocks completely replaced by silica. Banks (1970) studied the Leadville
Limestone cherts in central Colorado and concluded that the late "chert" of the Leadville, in part the
jasperoid of Spurr, is found largely along fossil aquifers. Banks also concluded that the post-lithification silicification
in the Leadville was contemporaneous with its uplift and karst formation during the Mississippian.
In the Aspen area the silicification of the. Leadville Limestone and the underlying Dyer Dolomite forms a discontinuous
rim around the edges of some fossil karst sinkholes at the contact of the massive unbroken carbonate rock and the
sinkhole-filling breccia (Maslyn, 1976). At the Castle Butte Sinkhole the silica replaced portions of the east
and west walls of the sinkhole altering it to a rusty-brown sugar textured quartzite from 2 to 14 ft across (Fig.
5). Similar features occur around at least three other major fossil karst sinkholes on Aspen Mountain. Around the
sinkholes the silicified carbonate appears as a white, red, and rusty-brown saccharoidal quartzite and functions
as a guide to the sinkhole's location and shape. Silicification is also present at the edges of the sinkhole exposed
by mining in the Down-Under mine at Lenado and includes the replacement of portions of the sinkhole breccia. Silicification
also occurs as fracture fillings near the top, sides, and base of the sinkhole.
A similar pattern of silicification of the Leadville Limestone - Ouray Dolomite interval is present in the San
Juan Mountains of southwestern Colorado (Maslyn, 1977). Here the silica encrusts the paleosinkholes in addition
to completely replacing portions of the carbonates. Silica rims are also present around some of the limestone breccia
fragments in the red Molas silt- stone overlying the Leadville.
A number of possible mechanisms have been suggested for the replacement of the carbonate by silica. In view of
the pattern of silicification along water courses in the various carbonate rocks, and in sinkholes which would
presumably have been areas of surface water inflow or accumulation, it seems likely that in this area the silicification
is a near-surface phenomena possibly related to the release of silica during soil formation (Maslyn, 1976). |
BRECCIA PIPES
Karst breccia pipes form when the collapse of a cavern roof propagates upwards through a thick section of the overlying
roof rock, stoping its way towards the surface. The collapse continues until the increased volume of the pipe breccia
completely fills the pipe and the void into which it collapsed. Landes (1945) reports breccia pipes extending through
more than 3,000 ft of rock overlying a cavern in soluble salt beds.
The wall rock enclosing the breccia pipe need not be a carbo- nate unit, and very commonly is not. The pipes are
commonly filled with a breccia composed of angular, variably-sized frag- ments derived from the rocks the pipe
has penetrated and a net movement downward of the fragments is evident. Breecia matrix material may include siltstone,
clay, unconsolidated rock fragments, or there may he no matrix at all.
Because of the pipe's vertical extent their bases are seldom exposed and hence the determination of a pipe's origin
is some- times difficult.
One area where several -fossil karst breccia pipes are ex- posed in cross-section is in the Terlingua, Texas, mercury
district (Yates and Thompson, 1959). There they have mapped 76 breccia pipes with up to 1000 ft of vertical extent.
Some of the pipes are exposed in cross-section where they are seen to bottom in collapsed caves in the Cretaceous
Devil's River Limestone. Pipe shapes range from circular to elliptical with both shapes occurring at different
levels of the same pipe. The breccia pipes extend up to 250 ft in diameter with near vertical or vertical walls
(Fig. 6). Breecia filling the pipe is composed of fragments derived from the rocks overlying the collapsed cavern.
The blocks are cleanly broken off, suggesting that they caved into the pipe bed by bed. The stratigraphic relations
indicate that some of the breccia may have dropped at least 500 ft. This amount is not surprising as modem vertical
karst shafts over 1000 ft deep occur in widely scattered areas of the world.
Within the pipes are fillings of calcite, analcite, and sometimes hydrocarbons. The latter are locally abundant
enough to cause the ovens processing the mineralized breccia ore to overheat.
POSITIVE KARST FEATURES
Karst features are most commonly thought of as topographically negative landforms, such as sinkholes and caves,
which occur below the land surface. Karst tends to be climatically reflective in its landforms, however, and under
some conditions sinkholes predominant in a karst area, coalesce, and leave what were intervening ridges as isolated
hills on a lowered land surface. Such hills, known as either tower or kegel (cone) karst, can extend up to 600
ft above the surrounding countryside, |
|
Page 6
|
|
|
Fig. 6 - Cross-sectional view of a fossil karst breccia pipe exposed at the 248 Mine in the Terlingua, Texas mercury
district. From Yates and Thompson(1959). |
|
Page 7
|
|
resembling pinnacle reefs complete with talus accumulations along their sides. Tower karst has near vertical or
vertical walls. Kegolkarst is an immature form of tower karst and the hills am more cone shaped.
Fossil tower karst has been described by Maslyn (1977) from the Molas Lake area of southwestern Colorado. There
three tower remnants up to 400 ft in length and 68 ft in height extend into the younger, overlying Pennsylvanian
Molas Formation from a base on the Leadville Limestone-Ouray Dolomite interval. Mantling the tower sides are two
fragmental units, a limestone conglomerate and a Molas-cemented karst breccia (Fig. 7). The breccia fragments in
both these units were solutionally and mechanically derived from the tower core and accumulated as talus along
the sides of the towers. The major difference between the two units is the breccia matrix; red Molas siltstone
in the karst breccia, and minor amounts of tan clay residuum in the limestone conglomerate,. Such positive karst
features, when submerged, become important sites of potential reef growth (Purdy, 1974) and may influence the aerial
distribution of carbonate rock facies (Dodd and Siemers, 1971) and their later contained hydrocarbon accumulations. |
SUMMARY
It is hoped that the above descriptions of several of the more common preservable fossil karst features will aid
in the recogni- tion of these features and their importance in the geologic record. With the applied understanding
such knowledge can lead to the discovery of additional hydrocarbon accumulations.
REFERENCES CITED
Banks. N. G., 1970, Nature and origin of early and late cherts in the Leadville Limestone, Colorado:
Geol. Soc. Amer. Bull., v. 81, no. 10, p. 3033- 3048.
Bryant, Bruce, 1971, Geologic map of the Aspen Quadrangle, Colorado: U.S. Geol. Survey Map GQ-933.
Burdon, D. J., and A]-Sharan, A., 1968, The problem of the paleokarstic Damman Limestone aquifer in Kuwait: Jour.
Hydrology, v, 6, p. 385- 404.
Campbell, N. P., 1977, Possible exhumed fossil caverns in the Madison Group (Mississippian) of the Northern Rocky
Mountains. A discussion: Nat. Speleol. Soc. Bull., v. 39, no. 2, p. 43-54.
Cloud. P. E., and Barnes, V. E.. 1946. The Ellenburger Group of central Texas-. Univ., Texas Pub. 4621, 473 p.
Dings, M. G., and Robinson, C, S, 1957, Geology and ore deposits of the Garfield Quadrangle, Colorado., U.S. Geol.
Survey Prof. Paper 289. |
|
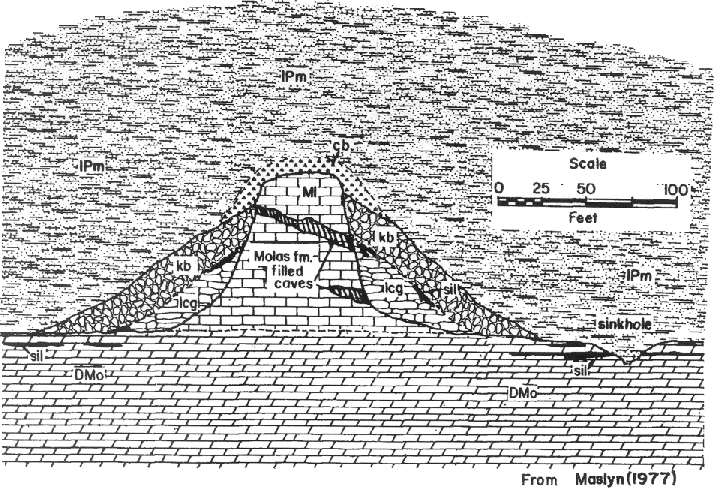
Fig. 7-Idealized cross-section of a fossil karst tower in the Molas Lake, Colorado area. The section includes limestone
conglomerate (lcg) and karst breccia (kb) resting on the Leadville Limestone (Ml) and the Ouray Dolomite (DMo). |
|
Page 8
|
|
Dodd, J. R., and Siemers, C, T., 1971, Effect of late Pleistocene karst topography on Holocene sedimentation
and biota, Lower Florida Keys: Geol. Soc. Am. Bull., v. 82, p. 211-218.
Ebanks, W. J., Euwer, R. M., and Nodin-Zeller, D. E., 1977, Mississippian combination trap, Bindley Field, Hodgeman
County, Kansas: Am. Assoc. Petroleum Geol. Bull., v. 61, no. 3, p. 309-330.
Hart, O. M., 1958, Uranium deposits in the Pryor-Big Horn Mountains, Carbon County, Montana, and Big Horn County.
Wyoming. United Nations 2nd Int. Conf. on Peaceful Uses of Atomic Energy, Geneva, Proceedings, p. 523-526.
Keefer, W. R., 1963, Karst topography in the Gros Ventre Mountains, Northwest Wyoming. U.S. Geol. Survey Prof.
Paper 475-B, p. B 129-B130.
Landes, K. K., 1945, The Mackinac Breccia: Mich. Geol. Surv. Pub. 44, p. 121-154.
Leith, C. K., 1925, Silicification.of erosion surfaces: Econ. Geol., v. 20, p. 513-523.
Maslyn, R, M.,. 1976, Late-Mississippian paleokarst in the Aspen, Colorado area: unpub. Colo. Sch. Mines M. Sc.
thesis, 96 P.
Maslyn, R. M., 1977, Fossil tower karst near Molas Lake, Colorado: Mountain Geologist, v. 14, no. 1, p. 17-25.
McCaleb. J. A., and Wayhan, D. A., 1969, Geologic reservoir analysis, Mississippian Madison Formation, Elk Basin
field, Wyoming-Montana: Am. Assoc. Petroleum Geol. Bull., v. 53, no. 10. p. 2094--2113. |
McKnight, E. T., and Fischer, R. P., 1970, Geology and ore deposits of the Picher Field, Oklahoma
and Kansas: U.S. Geol. Surv. Prof. Paper 588, 165 p.
Merril, W. M., and Winar, R. M., 1958, Molas and associated formations in the San Juan Mountains area, southwestern
Colorado: Am. Assoc.. Petroleum Geol. Bull., v. 42, no. 9, p. 2107-2132.
Quinlan. J. F., 1972, Karst-related mineral deposits and possible criteria for the recognition of paleokarsts:
A review of preservable characteristics of Holocene and older karst terranes: 24th Int. Geol.. Cong., section 6,
p. 156-167.
Richards, P. W., 1955, Geology of the Bighorn Canyon area, Montana and Wyoming-. U.S. Geol. Surv. Bull. 1026, 93
p.
Roehl, P. O., 1967, Stony Mountain (Ordovician) and Interlake (Silurian) facies. analogs of recent low-energy marine
and subaerial carbonates, Bahamas: Am. Assoc. Petroleum Geol. Bull., v. 51, no. 10, p. 1979- 2032.
Sando, J. E., 1974, Ancient solution phenomena in the Madison Limestone (Mississippian) of north-central Wyoming:
U.S. Geol. Surv. Jour. Res., v. 2, no. 2, p. 33-41.
Spurr, J. E., 1898, Geology of the Aspen mining district, Colorado, with atlas: U.S. Geol. Surv. Mon. 31, 160 p.
Walker, R. T., 1928, Deposition of ore in pre-existing limestone caves.. Am. Inst. Min,. Eng. Tech. Pub. 154, 43
p.
Yates. R. G., and Thompson, G. A., 1959, Geology and quicksilver deposits of the Terlingua district, Texas: U.S.
Geol. Surv.. Prof. Paper 312, 114 p. |
|
Page 9
|